The transition from traditional ICE to an EV World: Does it represent the biggest challenge to the Automotive industry?
Table of contents
Introduction
Much has been written on the topic of Automotive vehicle electrification and the anticipated transition from traditional Internal Combustion Engine (ICE) to Electric Vehicles (EV). The industry paradigm has moved on from the initial debate around if EV’s will gain market traction to focus on understanding how fast the transition will be?
The pace of transition has headwinds such as battery cost, range anxiety, charging infrastructure development and industry inertia from prior ICE technology investments and installed capacity. However, transition forces such as the potential for legislation (especially in Europe), EV subsidies, battery technology developments, and declining prices may also accelerate the transition. The transition is anticipated to vary significantly by geographic region, however, the global nature of the Automotive industry and associated supply chains will eventually drive a worldwide transition.
Battery technology, electronics, vehicle autonomy, changing value streams, and the disruption potential of new start-up OEMs have characterized much of the debate on EV’s. This article seeks to consider EV impacts on the body structure of the vehicle and highlight several topical technical and operational challenges presented by the transition to EVs.
Body Engineering technical challenges for EV’s.
Many early EV’s utilized body structures originally developed for ICE vehicles adapted to package the new electric powertrain, batteries, and associated modules. While this enabled the industry's 1st phase of EV development learning it highlighted the need for dedicated EV body architectures (and many other vehicle systems) more suited to the unique package, safety and platform strategies required to support optimization and scaling of future EV body structures.
One of the primary functions of the vehicle body structure is its role in crash safety. The structure provides the combination of strength and energy absorption characteristics required to resist and absorb impact energy in order to protect the occupants and key vehicle systems. The transition to EVs presents several opportunities and new challenges for EV crash safety development.
Crash safety
Simplistically crash safety development is a question of how to react and dissipate the kinetic energy of the crash event through the work done in deforming the vehicle body. The task is to minimize the forces on the occupants and critical vehicle systems due to deformation of the body structure and the dramatic deceleration experienced during a crash event. As engineers describe in the mathematical model, exhibit 1 below:

So, what is unique with EV’s that changes this crash model and the established countermeasures built from decades of ICE vehicle development? The formula above shows that the available crush space (Δ) and the efficiency of the crash structure (η) are key variables in managing crash energy (Together with the restraint systems). Controlling deformation and improving crush efficiency help minimize the potential deceleration and resultant injury risk for the occupant and key vehicle systems (such as the fuel tank with ICE vehicles).
The unique challenge for EV’s is the addition of the battery module and the need to protect the module from significant crush that may cause catastrophic failure. The challenge in many EV applications is the required size and location of the battery tray leaves very little crush space within which to react and absorb the impact energy, especially in side impacts. The Auto industries decades of ICE experience has provided an excellent understanding of how to protect occupants during side impacts leading to unified theories and significant convergence of side impact protection solutions. However, we now add into this challenge the need not only to protect the occupant, but also the battery module and likely with less available crush space within which to achieve this.
In contrast to the potentially more severe EV side-impact challenges front and rear crashes may provide opportunities to develop more efficient crash structures (η) and provide more crush space (Δ) due to the elimination of the gas tank and likely reduced package space required for EV motors v’s ICE engines, as illustrated in exhibit 2:
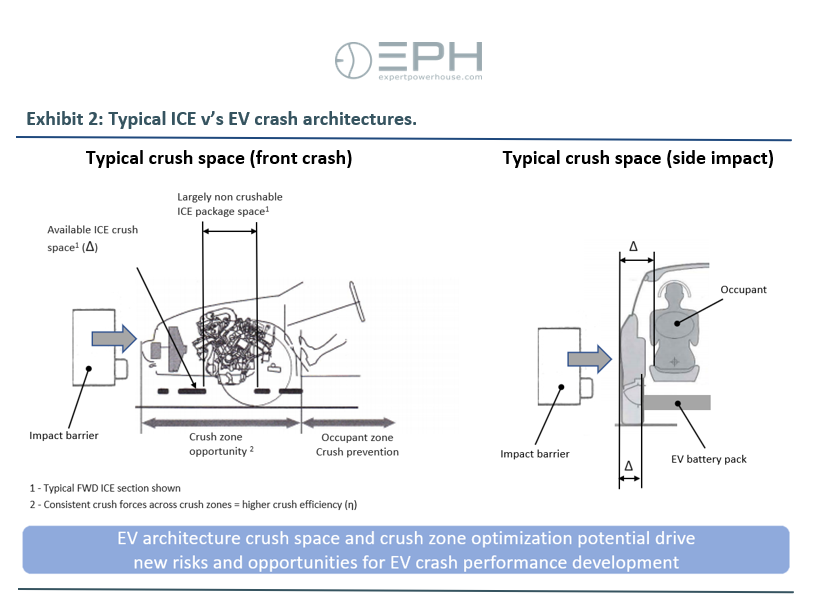
Reflecting this challenge, we are seeing intense activity in the areas of vehicle body side and Battery tray structure development such as:
- The emergence of a variety very high-performance rockers and body side reinforcing solutions in Steel, Aluminum, or multi-material combinations. The different material applications seeking to utilize the distinctly different material properties and available geometry options to provide the required combination of strength, stiffness and energy absorption characteristics needed to manage the impact energy.
- A wide variety of Battery tray design solution, material and manufacturing process solutions, each with significantly different cost, weight, and performance characteristics currently exists across the industry.
The multiplicity of solutions for EV battery trays and body side structure solutions evidences the lack of unified theories of best practice and resultant solution set convergence typical of mature technologies. OEMs and suppliers are seeking to address questions such as: Is it better to put energy management structure in the battery tray itself, in the surrounding vehicle body side and floor structure, or both? What architecture design, materials and manufacturing solutions offer the optimum cost, weight and performance balance for the customer, vehicle type and regulatory landscape? Ultimately what design, material and associated manufacturing process enables the optimum cost, weight, performance balance?
EV weight
This search for cost, weight, performance optimized body and Battery tray structure solutions for EVs raises a second key question for vehicle and body structure design. How should we think about weight in EV applications v’s ICE vehicles?
Contemporary body design has seen a significant focus on light weighting. Lower weight body structures provide an important contribution to improved vehicle performance and dynamics, however, it is emissions regulation that has driven the light weighting trends for ICE vehicles. In response to increasingly stringent emissions (or fuel consumption) regulation the industry has developed a thorough understanding of the various vehicle technologies that enable compliance with future regulatory targets. And importantly the relative cost (or value) of competing technologies such as engine stop start, low friction engines, aerodynamic aids, and light weighting. The relationship between solution cost and emissions reduction potential and weight and fuel consumption (and therefore emissions) is well understood. Such data has helped provide guidance as to the value of weight in ICE vehicle design and the development. Euro/kg (or $/lb.) cascaded cost targets provide a useful comparative measure to evaluate the value of lightweight technologies v’s alternative solutions. For mass market higher volume vehicle for example $2-4 /lb. has become a common target for affordable lightweight solutions (this varies by vehicle segment and specific program performance targets).
The question for EV’s is how to think about weight and the value of lower weight without the direct link to ICE emissions regulation. The beneficial effects of lower weight on attributes such as vehicle performance and dynamics still exist, however, in the absence of vehicle emissions what is lightweight worth (v’s alternative technologies) to an EV vehicle program?
Given the discussion of the potential impact of range anxiety for the adoption rate for EVs maybe range becomes the replacement weight consideration for EV applications. The traditional ICE paradigm that less weight = less energy required to move = less emissions (and improved secondary benefits) potentially becomes less weight = less energy to move = longer range for EVs. However, the EV model is more complex as the increased range that can be realized due directly to lower weight is relatively small (compared to the ICE link to emissions). This can be as little as 1 additional mile of range for a 10 kg weight save. The weight impact on range is further complicated by the potential of energy recovery technologies such as regenerative breaking.
Understanding the relative value of weight for EV’s especially v’s the rapidly changing cost of other technologies that can extend range (such a battery technology, motor and invertor technology, energy management applications, Aerodynamic aids etc.) is an important question for future EV vehicle chief engineers. Money spent on battery technology, motor and power management efficiencies can be more cost effective than the application lightweight materials, suggesting the prevailing ICE $/lb. benchmarks will reduce.
Multi-material skills
The journey towards the optimum cost, weight, performance body structure solution for ICE vehicles has seen challenges to traditional Steel body structures with the evolution of full Aluminum alternatives (mainly for premium vehicles) some composite solutions through to the prevailing paradigm that multi-material solutions (in proportions that vary by vehicle segment) provides the optimum solution.
For ICE vehicles (as noted above) the weight element of the optimization challenge was significantly incentivized by emissions regulation and the paradigm for EV application is redefining the optimum mix. Seeking lighter materials to avoid emissions regulatory penalties is being replaced by the need to find material combinations that address new EV requirements and potentially more severe safety load cases. And, in response to newer safety regulation such as small overlap, European MPDB and the anticipated more severe US side impact regulation.
The question for EV material selection becomes less about the weight but critically more about the need to thoroughly understand and consider multi-material solutions to utilize the very different strength, stiffness, and energy absorption characteristics of the various materials and associated manufacturing process affinities. For example, the challenging side impact requirements and associated battery pack protection needs requires both extremely high strength materials (Typically available with high and ultra-high strength steels) to resist crush and transfer impact energy together with the ability to absorb energy by deformation associated with lower grade Steels and Aluminum (which can also offer some geometry flexibility and lower volume manufacturing advantages). Therefore, the need to continue the multi-material journey incentivized by ICE emissions is likely to continue with EVs although with a different cost, weight, performance optimization motivation.
The EV multi material challenge is that although many Automotive companies have multi-material engineering understanding and capabilities, not all do. And the depth of understanding required for safety critical applications, associated early simulation and validation skills will require an even deeper understanding, including for:
- Advanced steels - Understand AHSS, UHSS and emergent Gen 3 steels the design and manufacturing integration challenges such as formability, welding, delayed fracture potential, corrosion performance and available coatings, achievable manufacturing process tolerances through to global material availability considerations.
- Aluminum application - Alloy application and development potential and cost drivers, extrusion and casting process understanding and associated tolerances, robust fracture prediction especially for emerging high strength grades, potentially material thermal and directional characteristics etc.
- Composite solutions – The various existing and emerging cost curves and volume affinities, directional property opportunities and challenges. Volume and scaling potential, failure prediction, composite material type and utilization cost impacts, ….. etc.
Engineering operational challenges for EV’s.
The transition from ICE to EVs has the potential not only to present new technical challenges for every component and system of the vehicle but can also impact the way we manage the New Product Development (NPD) process. EV challenges drive the need to adapt traditional ICE NPD processes, consider how we organize and collaborate and evaluate how the changing EV cost structures and emerging business models impact how we look at cost and value chains.
The new product development process
The transition to EVs has seen, and perhaps been driven in many areas by, the emergence of many new entrants into the segment. Fast moving new EV OEMs, without the inertia of installed capacity considerations and legacy ecosystems and responsibilities. Also, many new suppliers of advanced technologies new to the automotive space providing new services and business models that have the potential to restructure traditional industry value chains.
Both traditional and new OEM’s and suppliers are seeing the need to develop new capabilities that potentially impact how they develop and manufactures vehicles and components. For traditional OEM’s it is likely the need to acquire enhanced Electrical, Electronic and Software development skills and build new technology and service alliances. For established suppliers, the transition to EVs may significantly impact their traditional product offerings and in some cases negate the need for a company’s products and technologies altogether, presenting significant re-invention and change management challenges. For new EV OEMs, especially those emerging from a software / electronics background, it may be more about developing complete vehicle development skills, operating process and manufacturing capabilities or for technology suppliers perhaps how to interface with traditional OEM’s systems and processes.
Collaboration intensity
The Automotive product development process has traditionally been a very collaborative process. Requiring collaboration across functions within the OEM organization, across their supply base and on a global scale. The transition to EVs will place an even more demanding emphasis on collaboration across the new product development ecosystem, as:
- Traditional OEMs seek to work with new partners of advanced technology and services and potentially new EV OEMs to access new technologies.
- New OEMs will need to establish new supply bases and manufacturing capabilities either with in house investments or manufacturing partners.
- The investment intensity of new EV platforms will likely drive many players to seek to share the developments cost with joint platform, battery or manufacturing investments forming new development partnerships.
- Some OEMs will likely stop in house powertrain and transmission manufacture and switch to motor, battery and power management module purchase and therefore collaboration intensity and complexity will increase with this move from make to buy.
The NPD process used by OEMs and suppliers forms the essential “common language” to describe their vehicle development activities and is therefore a key enabler for collaboration and stakeholder alignment. The process describes the activities and importantly the timing and sequence of activities and decisions points required at each stage of development to robustly design, develop and launch a new vehicle. A high-level development model is shown below, exhibit 3, that is cascaded into many more departmental and summary stage gates and thousands of associated activities and associated responsibilities.
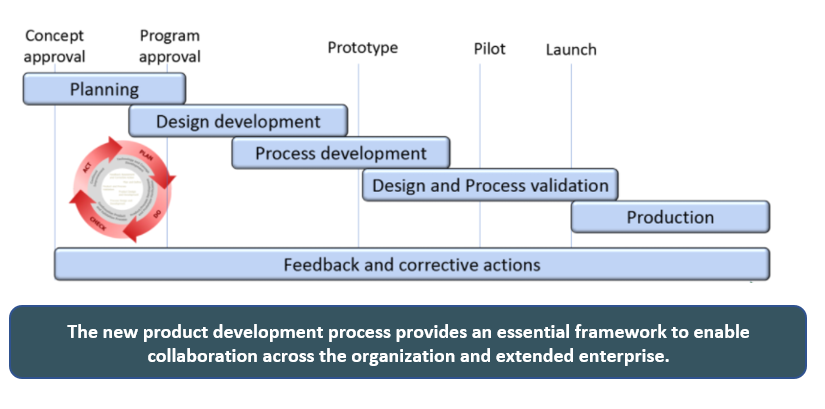
Establishing a well defined and commonly understood NPD process and specific vehicle plan provides the template to enable collaborators to understand, plan, report and track project progress and assure timely stage gate decisions (and timely identification of recovery activities if needed). The NPD process is essential to support lean, timely, product development activities and ultimately build confidence for a robust launch (Technically, Operationally and Financially).
Organization
The activities defined within the NPD process also describe an associated delivery responsibility and define or imply an associated organizational structure for the project. This framework (often cascaded to an appropriate level of RASIC chart) provides a useful, if not essential, framework to structure joint development activities, align stake holder expectation and potentially form the basis for legal contracts.
Planning and or role playing the development of a new EV systems or component through the anticipated development process can help identify gaps, development interdependencies and align stakeholders. For example, a new task for the EV development process is the design and development of the battery pack that raises questions such as:
- Perhaps obvious but to confirm which function within the organization owns the pack development and production release responsibility, or will it be split across functions? “Battery” functionality would likely identify an electrical department ownership. However, many EV battery packs (as discussed above) have very significant structural (crash) performance requirements that in most organizations are more likely found within body and or Vehicle Engineering functions (including the associated structural component material and manufacturing engineering heritage).
- The battery pack, if structural, will need to be developed within the overall crash safety strategy and resultant energy management approach for the vehicle, needing structure space that will compete with the essential energy density targets for the pack.
- The depth of skills for the battery, electronics and associated software development needs are unlikely to be found in body engineering organizations while complete vehicle attributes, such as thermal performance requirements, will need to be integrated into the overall vehicle development and validation activities.
While such cross functional development activity is not new to the industry the battery pack development is an example that will require an intensity of collaboration for a “new” vehicle module that may not be adequately described within existing functional NPD deliverable structure and RASIC. Role playing (or conducting a project pre-mortem) and detail planning review for the pack development can address key questions for the project and organization that can be addressed before they escalate into negative project cost, timing and or quality impacts.
IP landscape
For both traditional and new players in EV vehicle development activities understanding of the rapidly emerging IP landscape is also requiring a greater emphasis than more traditional new vehicle and especially refresh or platform derivate development. Considering body structure implications, the rapid pace and global diversity of EV solutions in the battery tray and safety structure space has seen the publication of numerous patents. Ensuring the periodic scanning and refresh of IP awareness with embedded milestones in new product development activities can avoid costly unintended infringements or help identify and capture valuable IP recognition opportunities.
EV cost structures
Characteristic of the transition from ICE to EV vehicles is the associated changes to the vehicle cost (and value) structure. The battery pack and associated power electronics component cost represent a huge relative increase in the vehicle component cost and can represent as much as 50% of the EV variable cost. Some manufacturing cost reduction is anticipated from the reduced complexity of EV motors and transmissions v’s complex ICE powertrains, but current battery cost more than negate such savings and with consumers reluctant to pay a significant premium for EV vehicles this potentially represents a significant impact to EV profitability. All components of the vehicle will therefore see an intense design to cost pressure.
In body engineering the performance element of the cost, weight performance balance is concerned with providing the required component or system combination of strength, stiffness, energy absorption and aesthetic characteristics that meets the required target performance. The emerging weight considerations were (at least in part) discussed above. The variables we have to play with to achieve cost targets are; The design choices we make (platform strategy, body architecture, through to detail section properties, joining methods etc.). The material selections we make (that can provide quite different strength, stiffness and energy absorption characteristics and cost), and the accompanying manufacturing process selections we make. Complicating this puzzle is that the cost side of the cost, weight, performance balance objective varies with volume, especially the manufacturing process selection.
With the difficulty of predicting volumes for EV adoption rates and specific vehicles (lacking historical ICE segment benchmarks) deciding upon and then optimizing the body structure solution for the target volume will become increasingly key, especially due to the investment intensive nature of body components and body assembly. The concept development phase of EV projects will require a careful and keen understanding of volume, material and manufacturing process affinities, economic break points, process volume sensitivities (and potentially feasibility, cost and timing for incremental capacity if required). For example, Aluminum extrusion intensive structures can be attractive for lower volumes as the higher material cost (variable cost) is more than off-set by the low tooling cost (capital cost) amortization. However, as volumes rise and especially if significant post extrusion forming and machining are required the rapidly escalating variable cost can make alternative (stamped, cast, roll formed, ….) solutions with higher tooling capital, but low variable cost a better option. Given the uncertainty of initial volume forecast volume flexibility will also become key and consideration given to the value of flexibility. E.g., does the chosen design lend itself to scaling of manufacturing automation during production, what are the capacity break points for the chosen tooling solutions?
The way we model costs and especially value in our vehicle attribute development mix may also need to be evaluated. Traditional vehicles describe the specific vehicle positioning (target market) with cascaded vehicle attribute development priorities and hence proportional spend or budget objectives. Valuing EV vehicle attributes will present challenges that come with new segments, how to value attributes (such as the weight example above) and potentially how best to embed considerations of the revenue generating potential of options especially for new services for EVs.
Summary
In summary the transition to EVs from traditional ICE vehicles will present not only technical challenges for all vehicle systems but also impact the engineering process itself, how we develop the skills and capabilities needed and how we organize and structure the supply chains and value streams. Some of the key body structure and engineering operations challenges (but not an exhaustive list) to be addressed include:
Safety (crash) performance
Developing and cascading unified theories for changing load cases, especially for Battery tray protection, newer and anticipated safety regulation
Weight understanding
Understanding the value of lightweight and the changing EV cost, weight performance balance v’s ICE and competing EV technologies.
New skills developments and partnerships
Including potentially new and deeper multi-materials development capabilities and associated global material supply consideration.
NPD process development needs
From integrating new load cases and materials for mature OEM’s through to developing engineering and operating models and standards for start-up OEM’s and suppliers. Enabling collaboration with mature NPD processes and developing the organization (and potentially global arrangement) of engineering teams and staying ahead of the emerging IP landscape.
Volume sensitivities
Especially given the potential volume forecast uncertainties and traditional investment intensity of body structures understanding manufacturing process volume affinities and breakpoints (and process capabilities) will be key to optimizing costs and managing volume ramp-up.
The transition to an EV world represents the biggest challenge to the Automotive industry in our lifetime. But this is what makes it one of the most exciting times for our industry and a time for reflection and reinvention!
Author: Roger Townsend, 35+ years of Global Automotive Engineering and Manufacturing experience together with award winning academics and customer endorsements. Major project delivery experience with Volvo, JLR, Ford, VW, GM, FCA, Honda, Nissan & Magna.